Ultrahigh Field MRI Group
Welcome to the ultrahigh field (UHF) MRI group at the BioMedical Engineering and Imaging Institute (BMEII) at the Icahn School of Medicine at Mount Sinai. Led by Dr. Priti Balchandani, the ultrahigh field MRI group devises creative engineering methods to overcome some of the main limitations of operating at high magnetic fields, thereby enabling high-resolution whole-brain anatomical, spectroscopic and diffusion imaging, as well as unlocking new contrast mechanisms and sources of signal. To achieve these goals, Dr. Balchandani’s team focuses on novel radio frequency (RF) pulse and pulse sequence design, and specialized hardware solutions such as parallel transmission. These techniques are used to improve diagnosis, treatment, and surgical planning for a wide range of neurological diseases and disorders.
In order to achieve these goals, Dr. Balchandani’s team focuses on novel radio frequency (RF) pulse and pulse sequence design as well as specialized hardware solutions such as parallel transmission. These techniques are ultimately applied to improve diagnosis, treatment and surgical planning for a wide range of neurological diseases and disorders.
Some clinical areas of focus for the team are:
● Improved localization of epileptogenic foci
● Imaging to reveal the neurobiology of depression
● Development of imaging methods to better guide neurosurgical resection of brain tumors
Epilepsy
Epilepsy adversely affects almost 3 million people in the United States. 15%-30% of these individuals do not respond to medication and may be candidates for surgical intervention. Due to excellent soft tissue contrast and high-resolution visualization of brain anatomy, magnetic resonance imaging (MRI) plays a vital role in the preoperative localization and characterization of brain abnormalities for patients undergoing epilepsy surgery. Ultrahigh field MRI has great potential to unearth subtle abnormalities in vivo that are undetectable at lower fields. At Mount Sinai, we have available to us a state-of-the-art research-dedicated 7 Tesla (7T) human MRI scanner to design and validate new imaging techniques and apply them to study disease. 7T MR is a powerful noninvasive tool to 1) increase conspicuity of epileptogenic abnormalities resulting in improved detection efficiency and reduced use of invasive electrophysiological evaluation and 2) provide more accurate delineation of lesion boundaries aiding in neurosurgical planning and leading to better patient outcomes. Unfortunately, multiple technical issues associated with high field operation, including inhomogeneity of the main magnetic and applied radiofrequency (RF) fields, result in artifacts for imaging and spectroscopy, limiting the utility of current high-field scanners. Our lab is designing innovative RF pulses and pulse sequences to overcome these limitations in order to fully utilize the signal-to-noise ratio and novel contrast mechanisms offered by high-field MR magnets for imaging and spectroscopy of epilepsy. These tools will be combined to compose a comprehensive 7T epilepsy imaging protocol. We are collaborating with neurologists Drs. Madeline Fields and Lara Marcuse to evaluate this protocol in a pilot study of epilepsy patients who are candidates for surgical intervention.
Psychiatric illness
Major depressive disorder (MDD) affects 6.9 % of the U.S. adult population and costs an estimated $104 billion per year. Despite this tremendous impact on society, the biological mechanisms of MDD are still poorly understood and consequently treatment options are limited and often ineffective. There is a clear need to establish the abnormalities in the regions and circuits of the brain associated with MDD in order to enable the development of more effective, biologically targeted treatments. Magnetic Resonance Imaging (MRI) can be used to measure various properties of brain tissue, however, current MRI methods possess insufficient resolution and sensitivity to capture many of the subtle, yet critical, changes that can serve as neurobiological markers for MDD. Ultrahigh field MRI scanners, such as those operating at 7 Tesla (7T), are now making it possible to noninvasively visualize smaller, more subtle abnormalities in human brain structure and metabolism. While 7T scanners are more able to detect fine changes in brain tissue than conventional lower-field scanners, there are several physical limitations and technical issues that prevent the benefits offered at 7T from being fully exploited. In this proposal, the goal is to overcome these limitations with the development of specialized MRI pulse sequences and novel radiofrequency (RF) pulses. From these technical developments a comprehensive, multimodal 7T MRI protocol will be built that can be used to establish imaging biomarkers for MDD. Specifically, the aims of this proposal are: 1) To develop high-resolution 7T structural, diffusion and spectroscopic imaging methods to reveal grey matter abnormalities, white matter degradation and cellular loss associated with MDD and 2) To build a multiparametric 7T imaging protocol and apply it to compare quantitative imaging measures between a group MDD patients and controls. The design goal for the proposed 7T imaging sequences is to achieve 20–40% greater signal-to-noise ratio in important brain regions, while remaining within safety limits. The hypothesis is that the high-resolution, multimodal imaging data obtained utilizing these methods will reveal grey matter volume reduction, white matter degradation and neuronal and glial loss in substructures of the hippocampus and medial prefrontal cortex in MDD patients when compared to healthy controls. These quantitative imaging biomarkers for MDD will have significant value in noninvasively assessing treatment response and tailoring new therapies based on the fundamental underlying biology of MDD. This will ultimately lead to the development of more targeted and effective treatments for MDD, enhancing the quality of life of the millions of people who suffer from this disabling disease. Furthermore, because they are developed to address fundamental problems in 7T imaging, the tools produced in this study will be generally applicable to high-field MRI imaging of the brain, and so could be used to improve diagnosis, treatment and neurosurgical planning for a wide range of other neurological diseases.
Brain tumors
Growing, erosive and infiltrative tumors of the skull base such as lymphomas, malignant sinonasal tumors, gliomas, pituitary adenomas, meningiomas, craniopharyngiomas and metastatic disease represent a large percentage of all intracranial tumors and pose some of the most complex challenges in neurosurgery. Not only is skull base tumor pathology difficult to treat, but the anatomic location of these tumors involves the most densely populated critical structures in the brain. Endoscopic endonasal surgery (EES) has transformed skull base tumor resection as it exploits safer, more direct, minimally invasive anatomical access routes to a large number of intracranial lesions. However, three major challenges still impede the use of EES. These are: 1) Injuries to blood vessels that are in close proximity to or encased within the tumor resulting in cataclysmic bleeding, 2) Accidental damage to the optic apparatus, including the optic nerve and chiasm and 3) Limited knowledge of tumor composition and boundaries, preventing safe and complete resection. Magnetic resonance imaging (MRI) at ultrahigh field strengths, such as 7 Tesla (7T), offers exceptional resolution and contrast and is particularly effective at elucidating nerves, vasculature, fiber tracts and tumor anatomy. We propose the first ever application of 7T multi-modal MRI to provide ultraprecise image guidance for endoscopic surgical planning and intra-operative decision-making. Our lab has the specific expertise in developing signal transmission tools, such as novel adiabatic radiofrequency (RF) pulses, to upgrade conventional imaging methods to robustly perform at ultrahigh fields. We hypothesize that our optimized 7T imaging techniques will depict tumor anatomy and adjacent structures in unprecedented detail, transforming pre- and intra-operative decision making for EES, and dramatically increasing the success rate, safety and applicability of the technique.
RF Pulse Design
The primary research goal of our lab is to develop anatomic, spectroscopic and diffusion magnetic resonance (MR) imaging tools to elucidate changes in brain structure, metabolism and connectivity in the presence of disease. Our work is focused on the application of signal processing principles to the development of novel radio frequency (RF) pulses and pulse sequences that maximize the information imparted by MR images. We have developed several techniques to harness the power of high-field MR magnets by overcoming physical and hardware limitations in order to visualize the human brain in unprecedented structural and metabolic detail. Together, these techniques have the potential to drastically improve diagnosis, treatment and monitoring of neurological diseases and disorders as well as advance our understanding of the brain in the normal state.
Hardware
Our main areas of hardware development are:
1) Designing custom coils to support research projects at TMII;
2) Exploring new coil design concepts and optimal design for individual applications and
3) Investigating local SAR in ultra-high field MRI with full-wave simulation of the RF system to ensure safety of subjects.
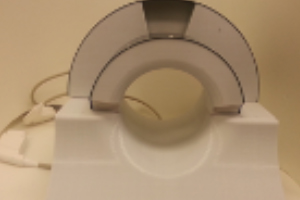
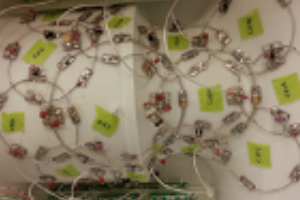
Team and Alumni
Priti Balchandani
As the Director of the High Field MRI program at BMEII, Dr. Balchandani focuses on developing novel techniques to exploit the power of high-field MR magnets to visualize the brain in unprecedented detail. She leads a team of 7T scientists to devise creative engineering methods to overcome some of the main limitations of operating at high magnetic fields, thereby enabling high-resolution whole-brain anatomical, spectroscopic and diffusion imaging as well as unlocking new contrast mechanisms and sources of signal. In order to achieve these goals, Dr. Balchandani’s team focuses on novel radio frequency (RF) pulse and pulse sequence design as well as specialized hardware solutions such as parallel transmission. These techniques are ultimately applied to improve diagnosis, treatment and surgical planning for a wide range of neurological diseases and disorders. Some clinical areas of focus for Dr. Balchandani’s team are: improved localization of epileptogenic foci; imaging to reveal the neurobiology of depression; and development of imaging methods to better guide neurosurgical resection of brain tumors.
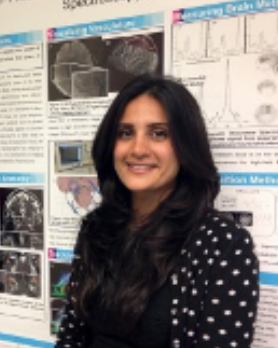
Akbar Alipour
Dr. Alipour obtained his PhD degree in Electrical and Electronics Engineering, with a focus on MRI from Bilkent University, Ankara June 2017. In November 2017, he joined the Johns Hopkins University School of Medicine and Biomedical Engineering as a postdoctoral fellow working on the development of cardiovascular devices for interventional MRI applications. He moved to Mount Sinai School of Medicine, Biomedical Engineering and Imaging Institute in August 2019, continuing his research on parallel transmission, developing pulse sequences, RF safety, and sodium imaging at UHF MRI Prof. Priti Balchandani’s lab.
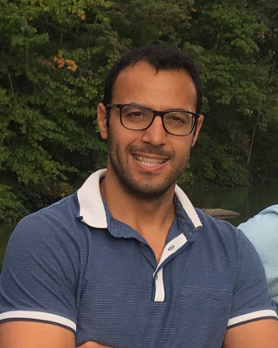
Alan Seifert
Alan is currently an Assistant Professor of Radiology in the Biomedical Engineering and Imaging Institute. He received his B.S. in Biomedical Engineering from Johns Hopkins University and his Ph.D. in Bioengineering from the University of Pennsylvania, and completed his postdoctoral training at Mount Sinai. His research is focused on technical development and translation of functional, diffusion, high-resolution anatomical, and quantitative myelin density MRI methods in the human brainstem and spinal cord. Using these methods, he studies the structure and function of neural circuits that modulate the sensitivity to pain, and he is working to develop imaging biomarkers that can aid in the development of non-pharmaceutical interventions for pain relief.
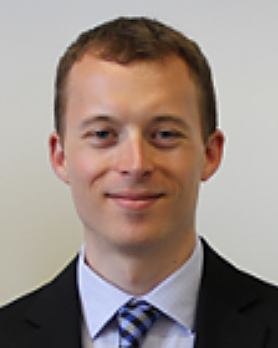
Francesco Padormo
Dr Francesco Padormo obtained an MSci in Physics (2008) and PhD in Magnetic Resonance Physics (2012) from Imperial College London. He then became Research Associate at King’s College London, working in collaboration with the University of Oxford on Ultra-High Field MRI, Parallel Transmission and RF Safety. In 2016 he moved to the Translational and Molecular Imaging Institute at Mount Sinai Hospital, New York City, continuing his research in the lab of Prof. Priti Balchandani, developing pulses sequences and methods for UHF imaging of skull-base tumors.
Gaurav Verma
Dr. Gaurav Verma is a postdoctoral fellow at the Icahn School of Medicine at Mount Sinai specializing in the development of accelerated and highly-sensitive spectroscopy and imaging sequences for ultra-high field MRI. He has implemented these sequences in the study of human gliomas, psychiatric disorders such as major depression and schizophrenia, and neurological disorders including amyotrophic lateral sclerosis, multiple sclerosis and epilepsy. Dr. Verma’s ongoing work explores novel imaging sequences to characterize highly sensitive imaging and metabolic biomarkers for neuropsychiatric disorders, and image processing techniques employing conventional & machine learning based strategies to glean additional data from the acquired images.
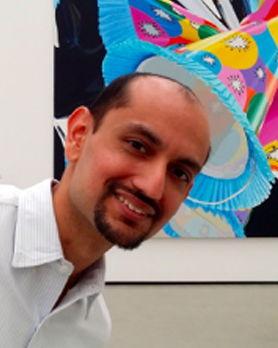
Jack Rutland
Jack Rutland graduated from Wake Forest University in 2016 where he earned his BA. He then moved to New York City to join Dr. Priti Balchandani’s Field Field Neuroimaging laboratory in the Translational and Molecular Imaging Institute at Mount Sinai Hospital. Jack’s research focuses on advanced imaging biomarkers of certain neurological diseases such as epilepsy and major depressive disorder. Jack will matriculate at the Icahn School of Medicine at Mount Sinai in August of 2018 and will continue to work in Dr. Balchandani’s lab during medical school.
Judy Alper
Judy Alper graduated with a BA in Physical Sciences (2014) from Yeshiva University and in 2015, while pursuing a MSci in Biomedical Engineering at City College, she joined Professor Priti Balchandani’s High Field Neuroimaging lab at the Translational and Molecular Imaging Institute at Mount Sinai Hospital. She transitioned from a MSci to a PhD in 2016. Judy’s research focuses on development of spectroscopic pulse sequences and image analysis for detection of imaging biomarkers for neurological disorders such as trigeminal neuralgia and major depressive disorder.
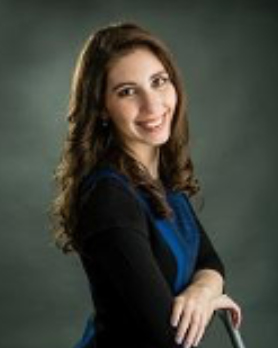
Laurel Morris
Laurel obtained her Ph.D. in Psychology from the University of Cambridge, UK. Before that she received a Bachelors in Pharmacology and Neuroscience from Nottingham Trent University and a Masters in Neuroscience from University College London, UK. Her work includes testing high-resolution neuroimaging protocols for measuring neural circuit organization, building cognitive models for complex neuropsychiatric disorders and exploring clinical psychopharmacology related to depression and anxiety.
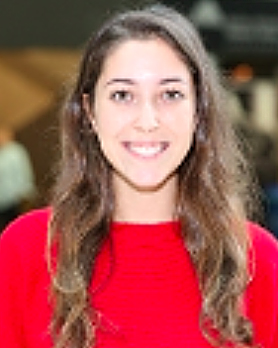
Yael Jacob
Dr. Yael Jacob’s research focus is developing and implementing new computational methods for the study of complex brain networks using functional and structural MRI. The main goal of her research is to understand how brain networks work and how different networks or sub-networks communicate and influence each other during different mental states. Dr. Jacob specialize in the mathematical field of graph theory and use it to study how neural networks interact during different cognitive tasks and their different manifestation among brain pathologies. She had implemented these methods deciphering brain network mechanisms that underlie behavioral and pathological differences in different neurological diseases and psychiatric disorders. Her current work is focused on applying graph theory and machine learning algorithms to decipher imaging biomarkers of certain pathologies such as epilepsy and major depressive disorder.
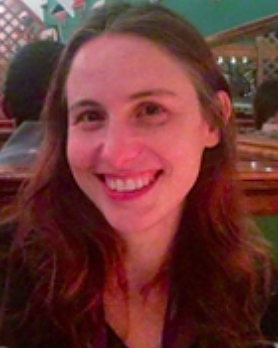
Rebecca Feldman
Rebecca Feldman’s research focus is on the development of high-field magnetic resonance imaging and magnetic resonance spectroscopy pulse sequences. Her goal is to use these technical developments to unlock the potential of 7T MRI to examine the structures of the human brain in extraordinary detail. In her spare time, Rebecca adapts 7T MR images into beautiful representations of the brain. Prior to joining Dr. Balchandani at TMII, Rebecca worked developing 23Na-MRI sequences at the University of Alberta. She has an MSc and PhD in Medical Biophysics from the University of Western Ontario, and a BASc in Engineering Science (Electrical) from the University of Toronto in Canada.
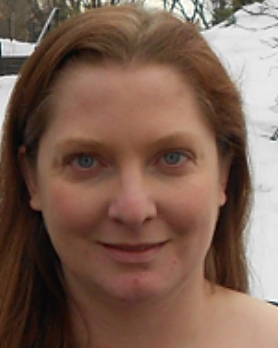
News
[VIDEO] Aspen Ideas Festival: Dr. Fuchs and Dr. Fayad on Powering Medicine with Technology and Data Science
Zahi A. Fayad, PhD, Director of Biomedical Engineering and Imaging Institute sits down with Thomas Fuchs, DrSc, Dean of Artificial Intelligence and Human Health to discuss powering medicine with technology and data science. Click the image to watch the video.
[VIDEO] Dr. Zahi Fayad: Powering Medicine with Technology and Data Science
BMEII Director Zahi Fayad discusses the role of data science in medicine at the 2022 Aspen Ideas Festival. Click the image to watch the video.
Latest Publications
Artificial intelligence–enabled rapid diagnosis of patients with COVID-19
Xueyan Mei, Hao-Chih Lee, […] Yang Yang
Nat Med (2020).
Probing myeloid cell dynamics in ischaemic heart disease by nanotracer hot-spot imaging
Max L. Senders, Anu E. Meerwaldt, ... Willem J. M. Mulder
Nat. Nanotechnol. 15, 398–405 (2020).